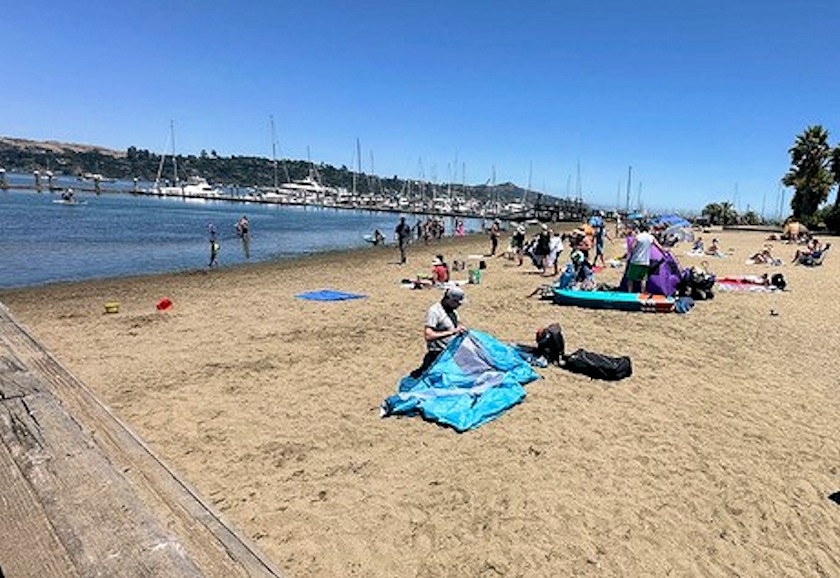
There is a beautiful little beach in the middle of Schoonmaker Marina. On sunny summer days you see children and parents lying on the sand and splashing in the water, maybe even a few serious swimmers [Fig. 1]. But typically, several times a year you will find the beach empty except for a few signs stating that the water is unsafe for swimming due to presence of coliform bacteria in the water. Who put the signs there, are coliform bacteria really that dangerous, and where did the bacteria come from?
During the warmer months of the year Marin County Environmental Health Services samples the water at beaches along our shores every other week and spreads a sample on plates of agar containing a nutrient broth, then puts the plates in an incubator at 37˚ C (98˚ F, our body temperature) overnight. If a significant number of colonies are seen on the plate, the beach is declared unsafe and marked with warning signs.
Are coliform bacteria that dangerous? Not usually, most species are harmless. They grow in the colon, the lower part of the intestine of warm-blooded animals. A large fraction of feces is living and dead coliform bacteria. Their presence thus implies that the water is contaminated with feces and thus could contain harmful bacteria and viruses. They are an indicator species that warns us of possible danger.
Usually, the coliform come from broken sewer pipes near the shore. Shorelines that are made of fill, which includes the Marinship area, Strawberry, and North Belvedere, are unstable and shift with time, rain, and heavy truck traffic. Clay sewer pipes are of course easily broken, but even newer plastic pipe will crack under these strong stresses. Anchor-outs are often blamed, but usually don’t produce the volume of sewage to register in these tests. We have two sewage treatment plants in this area, one near Yellow Bluff and the other at the end of Pickleweed Inlet. However, they are well monitored, and the outfalls are out at the edge of Racoon Strait.
If no bacterial colonies appear on the plate, does that mean that sea water has no bacteria? No, although until about 1960 the oceans were thought to be sterile for that reason. Then fluorescent stains for DNA were developed and sea water treated with these stains was seen to contain a large number of small fluorescent objects. These objects were found to be bacteria that didn’t grow at 37˚ C on agar plates. We know now that there are also viruses in the ocean, but they prey on marine bacteria, not humans.
The power of the exponential
An exponential is the result of repeated multiplication and has the notation ne, meaning n multiplied by itself e times. Thus, 210 is 2 times itself 10 times, or 2 to the power of 10, which is about 1000 (1024). When a bacterium lands on a culture plate at 37˚ C, it grows exponentially, typically doubling every 30 minutes. In 5 hours, or 10 doublings, there are about a thousand cells, in 10 hours a million; the number increases exponentially with time [Fig. 2]. Even though bacteria are small, a colony of a million is visible as a tiny dot. In 15 hours, there will be a billion cells, which is a large colony. However, bacteria native to the ocean grow very slowly at typical ocean temperatures of around 10˚ C (50˚ F) and may not grow at all at 37˚ C.
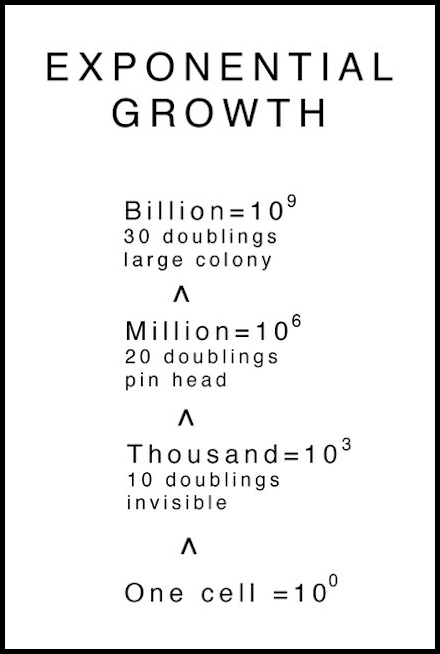
Now that we know how to look for them, bacteria have been found almost everywhere. In the ocean, rivers, lakes, soil, and hundreds of feet deep in the earth in mines and well holes. In fact, the only limiting factor seems to be temperature. Temperature increases with depth in the earth, and bacteria can’t survive at a temperature much above 120˚ C, (about 5 km deep). Most of these bacteria have not been cultured because we don’t know their nutritional requirements and they grow so slowly that it’s difficult to do much work with them anyway. What we now know comes mostly from their DNA.
Sequence baby, sequence
When the structure of DNA was predicted in 1953 by Watson and Crick it marked the end of a long scientific and philosophical journey from vitalism to chemistry. Before 1828 it was believed that all chemicals made by living organisms were special, or organic. They were a unique expression of a life force, typically associated with a mystical God, and could not be made artificially by man in the laboratory. The synthesis of urea in that year by Wohler was the first major blow to this idea of vitalism, that life was special in some fundamental sense. Year by year, scientific research revealed increasingly complicated molecules and chemical reactions in living organisms. But the story always ended at the step where new life was formed; how are characteristics of the parent transmitted to children? Are genetic traits coded by chemicals or are there other mysterious agents? Watson and Crick didn’t really care about the structure of DNA, but they guessed that the structure would reveal the mechanism of heredity, the code for genetic information and the process for transmission of this information to progeny. Their bet paid off big time! We now understand how the sequence of nucleotides (or bases) that make up the incredibly long strands of DNA in the nucleus of our cells, codes for the protein machines that power living organisms. Exactly what all the machines do, however, is a work in progress.
But here we are not interested in the philosophical importance of DNA, but its use as a tool in biology and medicine. In 1983, Kary Mullis, then an unknown chemist, was working at Cetus, a biotechnology corporation in Emeryville, CA (where I also happened to work at the time). Proving that genus can appear in unexpected places and times, Kary invented the polymerase chain reaction (PCR), which can exponentially replicate (thus “chain reaction”) a DNA sample, doubling the number of molecules every 1-2 minutes. Using PCR, a DNA fragment from bacteria or virus particles can be amplified (replicated) much faster than the organism could grow. PCR is a powerful tool to examine organisms like marine and soil bacteria, where the conditions for growth are not even known. Mullis was awarded the Nobel Prize for his work in 1993.
PCR requires a DNA primer, a small segment of DNA with a sequence complementary to some part of the DNA you wish to amplify. This requirement can be a virtue because it means that the amplification can be tailored in specificity to the organisms you are looking for. Some viruses, e.g. COVID, have RNA genomes, but there are enzymes that can produce DNA copies of RNA, so PCR can also be used for them also.
Today we have incredible instruments for determining the base sequence of DNA. As just one example, the MinION™ from Nanopore Technologies sells for $2,000, is powered by a laptop, and reads 400 bases/sec. There is a backpack model that can be used in the field. Since the COVID genome is 30,000 bases long, a virus specimen can be read in about a minute once the sample is prepared and loaded into the device. A bacterial genome would take a few hours to sequence completely, but usually only a partial sequence is required. Tools like these allow us to follow the evolution of COVID mutants in real time during an epidemic.
A dramatic example of searching the ocean for marine bacteria was the voyage of Sorcerer II, the large sailing ship owned by Craig Venter, the leader of one of the groups that produced the first draft sequence of the human genome. Across thousands of miles in the Pacific and Atlantic Oceans water samples were taken and the marine bacteria cataloged using PCR. However, for just seeing if there are coliform bacteria in our Bay it’s easier and cheaper just to spread a little water on a petri dish.
Life and death in the Bay
Richardson Bay is full of life. A few of the animals, plants, and algae have been described in these pages. But every living thing dies, and most lifespans in the Bay are brief by human standards, days to months. The living population of the Bay can change within months or even weeks when temperature and sunlight change, because of the rapid turnover of its life. In addition, living organisms constantly dump trash in the Bay. Crustacea shed their hard outer shells as they grow in size, molt, and finally die [Fig. 3]. Animals defecate undigested food after it passes through their intestines. But corpses and trash don’t seem to pile up, mostly because they are recycled by bacteria. Bacteria will eat almost anything. Plastics can degrade our environment so badly because they are most of that almost. Plastics just pile up, even though they may be physically broken into micro-plastics, maybe the most dangerous of all.
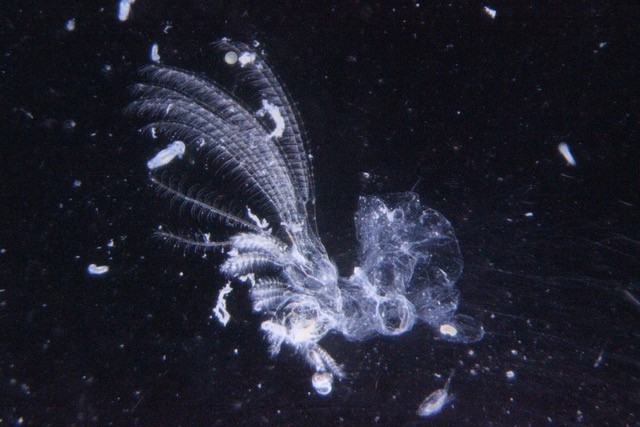
While some bacteria are suspended individually in the water, most form the outer layer of larger, decaying organic fragments. Individual bacteria and virus particles are also rare in the air, most are bound to dust or aerosol particles, which is why HEPA filters and masks are effective.
Who eats bacteria?
Even if most bacteria in our Bay are part of larger organic fragments, most of these are still small. Who eats them? The filter feeders do. The ultimate filter feeders are the clams, mussels, and oysters who pump water across internal sticky, mucus covered structures. Cilia then move the food into their stomachs. Increased turbidity of water in bays and estuaries after destruction of shellfish beds has been extensively documented. Clear water returns if the beds are restored. The fact that oysters are filter feeders means that lovers of raw oysters need to be mindful of their origin or a lot of bad bacteria will end up going down their throat.
Our deep history
Our earth is about 4 billion years old, but the young earth was quite different than the one we live on now. For 1-2 billion years our atmosphere was reducing, containing only nitrogen, carbon dioxide, and probably ammonia. Then, cyanobacteria evolved from some unknown ancestor and were able to use sunlight to split hydrogen from water, releasing oxygen into the atmosphere causing the Great Oxidation Event. This enabled fish, plants, you and me, to evolve and live in our present oxygen containing atmosphere.